Analytical considerations for cellular therapy manufacturing
Cell Gene Therapy Insights 2016; 2(6), 651-661.
10.18609/cgti.2016.071
The commercialization of cell-based products presents unique challenges. An evolving regulatory landscape, rapid advances in manufacturing technologies, products with complex mechanisms of action (MOA) and inherent variability in starting cellular material are just a few examples of these challenges. The availability of relevant analytical tools is important in manufacturing and process development, where they are used to control the product and to enable process changes. When considering process changes, cellular therapy manufacturers seek to apply sound engineering principles, develop good process understanding and use meaningful analytical methods for product characterization. The diversity and complex nature of cell therapy products requires an increasingly broad set of analytical tools to assist in this endeavor. Scientists engaged in assay development are challenged to select the most appropriate methods and adapt these assays to a quality environment. Cell and patient variability, multiple MOAs, lack of reference materials and complex analytical methods and instruments underlie some of the technical difficulties. This article discusses the testing strategies used by cell therapy manufacturers throughout the product development lifecycle as well as solutions to commercial challenges such as method validation, automation and potency assay development.
The Role of Assays in Cellular Therapy Bioprocessing
Analytical methods are essential tools in cell therapy manufacturing, for control of the manufacturing process, raw materials, intermediates and the final product. Assays are typically conducted in a Quality Control (QC) laboratory, following defined standard operating procedures (SOPs) and with a goal of demonstrating product safety, identity, purity, content and potency. Analytical methods are also critically important in process development, where they are used to support manufacturing investigations, characterize processes and enable process changes. In both of these settings, a broad set of tools is needed to build the process understanding and product knowledge necessary to advance products through to the clinic.
Selecting assays for process development can be challenging, partly because of a live cell’s complexity, but also because the critical quality attributes for most cell therapies are poorly defined and vary from product to product [1]. For these reasons it may be necessary, initially, to develop several assays, each measuring unique physical and biological characteristics of the product. The goal of this exercise is to identify metrics that are both biologically meaningful and sensitive to variations in the process. Process alterations may be unanticipated, as is often the case during a manufacturing excursion, or deliberate, as in process development experiments. While the task of developing many assays can be time and labor consuming, it fits nicely within the standard drug development model. As a product advances through clinical trials, process understanding, knowledge of the product and how it works are expected to increase. Concurrently, the manufacturing process and test methods are expected to evolve. Cell therapy manufacturers will look to simplify the manufacturing process, increase quality and capacity and reduce the overall cost of goods. Similarly, as a product’s critical quality and process characteristics become more clear, assays will be refined and improved, new assays will be identified and less informative assays will be eliminated. Today’s cell therapy products and manufacturing processes are likely to be considered crude in comparison to what we will see in the next 5–10 years [2]. Using a broad variety of assays throughout development, should facilitate the natural evolution of these products and ultimately make them more commercially viable.
Selecting Assays for Process Development & Manufacturing
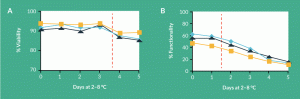
Figure 1. Selecting stability indicating assays: (A) cell viability and (B) cell functionality. The stability of a cellular product during storage at 2–8°C was assessed using a cell viability assay (1A) and a complex co-culture cell functionality assay (2B). Cell viability declined after 3 days of storage. Cell functionality dropped steadily after only 1 day at 2–8°C.
The selection of test methods depends largely on the questions that are being asked. A primary consideration when choosing an assay, for any purpose, should be its intended use. The requirements of a research method, for instance, may be limited to the relevance or identity of the analyte of interest and availability of the appropriate instruments. Assuming the correct controls are in place, it is not uncommon, or necessarily inappropriate, to draw conclusions from a research assay without investing much time in assay development. In the case of process development, changes are frequently investigated by varying a set of parameters within a single experiment. These types of studies, which are internally controlled, may also lessen the need for detailed assay characterization. A product release assay, on the other hand, is dramatically different. Desirable quality attributes for a release assay may include specificity, sensitivity, quantitative ability, accuracy, precision and robustness over time. As a product nears commercialization, several practical considerations will become increasingly important, such as ease of use, throughput, hands-on time and cost. The time and expense needed to characterize an assay destined for the QC lab is substantial, particularly for novel cell therapy methods. Test method validation, a requirement for regulatory approval, is similarly costly. Validation efforts require significant cross-functional interactions between development, quality and regulatory teams and close coordination with governing agencies. For these reasons, particular care should be taken to select the most appropriate assay before deciding to validate.
Diversity in the types of cell therapies, clinical indications, manufacturing processes, source material and assay technologies are a few of the factors that influence the choice of analytical methods. This variety is a main reason for the lack of standard assays in the cell therapy field. When deciding on a method, it is important to consider two factors, what to measure and how best to measure it. These decisions are context dependent and, as described above, depend largely on the assay’s intended use. The analytical scientist can choose measurements from a wide assortment of cellular properties, including physical (cell number, size, morphology), biochemical (viability, growth, metabolism, gene and protein expression) and cellular responses (differentiation, apoptosis, secretion, antigen recognition and cytotoxicity). The choice of measurement systems and instruments can also have a significant impact on the work required to characterize and ultimately validate an assay. Platforms such as the enzyme-linked immunosorbent assay (ELISA), for example, are commonly used, well understood and frequently validated for many biologic drugs. The development path for these types of methods is relatively straightforward. Flow cytometry has many applications in cell therapy development, from cell sorting, identification, characterization, functional testing and translational research. This technology, which is a staple in the field, is complex and, outside the transplantation field and early phase clinical trials, has been used mainly in research labs. As a result, the effort required to develop a well-controlled flow cytometry assay can be significant and should be considered carefully.
One strategy when choosing an assay might be to identify the simplest measure that can provide the necessary information. The pros and cons of this approach are highlighted in Figure 1. In this example, the stability of a cellular product was assessed using two different analytical methods. Cell viability displayed a decline after 3 days of storage (Figure 1A), whereas cell functionality dropped steadily after only 1 day at 2–8°C (Figure 1B). Because of its superior sensitivity, the cell functionality assay is preferred as a stability indicating method. When choosing between these tests, however, one also needs to consider the unique requirements of a stability assay. Specifically, stability assays must be capable of being validated. In making this decision, the complexity of the assay should be weighed. In this example, cell viability was measured by a simple membrane integrity test. Cell functionality, on the other hand, was assessed using a complex co-culture method. In contrast to the viability measurement, which was rapid and very precise, the functionality assay was lengthy, difficult to control, required careful operator training and displayed significant day-to-day variability. The validation paths for these two assays are likely very different. For the more complicated test, the analytical team must determine what additional work is required in order to successfully validate and control the method.
Measurement Assurance & Assay Validation
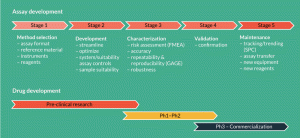
Figure 2. Assay development and validation using Quality by Design principles. Overview of the Quality by Design lifecycle for a cell therapy analytical method. In early clinical development, focus is on selecting the best analytical tools, including reagents, instruments and identifying appropriate assay controls. As the product advances in the clinic, detailed characterization of the analytical method is carried out. Risk assessment is used to identify potential assay robustness factors and guide experimentation. Statistical-based methods such as design of experiment and GAGE repeatability and reproducibility can be used to assess method performance in an efficient and organized manner. This rigorous approach will ease method validation and help develop tools for monitoring the assay throughout its lifespan.
Since most cell therapy assays are not widely used in the pharmaceutical or biologics industries, their validation paths have not been well defined. The heterogeneity of a cellular product, variability of manufacturing processes and a cell’s inherent complexity make it particularly important for cell therapy manufacturers to have confidence in their analytical measurements. As the number of cell therapies entering late phase trials grows and companies begin to seek regulatory approval, assay validation packages will be closely scrutinized. This underscores the importance of developing a comprehensive approach to analytical method validation.
When designing an assay validation strategy, it is important to recognize that assays share many of the same characteristics as a manufacturing process, in that they are influenced by both controlled (instruments, reagents, time, temperature) and environmental (operators, ambient temperature, reagent quality) variables. The common way of testing the impact of these factors on an assay’s performance is through a series of one-factor-at-a-time experiments. This type of experimentation is usually carried out during method development with a small number of high impact parameters retested during validation. In reality, however, most variables do not influence an assay in isolation. Instead, they are expected to interact, making it important to study combinations of factors. Addressing this reality during validation will create a more complete picture of assay variability and improve control over the method.
While this intricacy is generally understood, many analytical teams continue to treat assay validation as a box-checking exercise. This can be detrimental given the many inter-dependencies, time and cost associated with the effort. One way to streamline validation, and concurrently produce an increased understanding of the assay, is by applying quality by design (QbD) principles to the method validation process. QbD, which is encouraged by regulatory agencies, emphasizes risk assessment, planning, scientific rationale and empirical testing to help guide the generation of product and process understanding [3,4]. The technique, however, is infrequently used in assay development. An overview of the QbD process, adapted for an analytical method, is shown in Figure 2. QbD has an added value over traditional validation approaches in that it can help to prioritize experiments and assist in the development of methods in a phase appropriate manner. This will guard against over-investing in assay validation and increase the odds of success.
Fit-for-purpose is a commonly used phrase when discussing method validation [5]. The concept maintains that the level of validation should be tailored to an assay’s intended use. A detailed understanding of reagents, for example, may not be required for an assay that will be used in a single study. The term fit-for-purpose, however, does not imply that a validation only needs to be good enough. Assay fit-for-purpose should be addressed early in development. This will avoid spending time developing a test that has limited utility. Consideration should be given to the assay format, availability of reagents, controls and the complexity of instruments. The assay should then be streamlined to minimize lengthy or unnecessary steps and a mechanism for tracking the performance of the assay over time should be identified. Tracking and trending, if put in place early in the method’s lifecycle, will provide insight into real-life variables and serve as a basis for more thorough assay characterization. An important aspect of a method’s fit-for-purpose is its usability by the QC lab. Some assays, because of their complexity or lengthy turnaround time, may be less desirable from a QC perspective. This is particularly true for bioassays, which are inherently variable because they require extended culture periods, multiple (variable) cell types and generate more than one readout. While at times it may be necessary to validate a complex bioassay, it is worth considering whether there is something easier to measure that can provide the same information. Using several assays during early development may help to identify orthogonal methods that may be more straightforward to validate.
Qualification should be carried out before a test is used in a GMP environment. The criteria vary, but in general, assay qualification differs from validation in that there are no pre-set acceptance criteria and robustness is not studied in detail. Understanding an assay’s critical quality attributes (i.e., sensitivity, accuracy, precision, etc.) is required to assess whether it is capable of reliably performing its intended task. In advance of qualification, or validation, a risk assessment can be used to flag variables that might have a significant impact on the assay’s quality characteristics. The choice of variables should be based on the knowledge available at the time, experience with similar methods and include input from both development and operations teams. The experimental characterization of parameters identified in a risk assessment should be prioritized and documented throughout clinical development. Factors that have a significant impact on method performance will be interrogated before validation and may need to be controlled during routine use.
Generating the information needed to successfully validate an analytical method is a continuous and iterative process. Assay characterization should aim to better understand the impact of deliberate variations in the assay process on the assay’s critical quality attributes. Before starting, it is important to select suitable assay controls. Cell controls should display characteristics that span the range of outputs that will be measured by the assay. For example, if an assay will be used to measure cell viability, the corresponding cell controls should exhibit varying levels of viability, from low to high. While this may be challenging, particularly in early development, cell controls should be made in sufficient supply so that they can be used across multiple assay characterization experiments.
An organized statistics-based approach such as Design of Experiments (DOE) can simplify experimental planning, reduce the number of tests and deepen the understanding of variables that impact an assay process [6]. In early assay development, studies should focus on high-risk variables such as operators, instruments and key incubation times. Several robustness parameters can be tested in parallel using a screening DOE such as the Plackett–Burman. These multi-factor screens will identify the most important variables impacting assay performance and can serve as the basis for future characterization studies. The main effects found in a screen are subsequently explored in greater detail using more appropriately powered DOEs that focus on defining and then optimizing the assay process. Using this approach, information about the assay and how it performs under different experimental conditions will grow over time. This makes it easier to tailor the level of assay development to the product’s stage in the clinic. Understanding the impact of many factors, alone and in combination, on the method’s design space will ease the selection of assay acceptance criteria and set control limits for monitoring the method during QC use. Importantly, the higher level of knowledge gained using this approach will significantly increase the probability of a successful validation, provide tools for tracking method performance, assist in troubleshooting and facilitate assay transfers.
Commercial Challenges
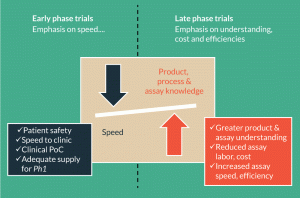
Figure 3. Assay considerations for early and late phase clinical trials. The focus of cell therapy developers is different for products in early versus late phase trials. In early development, attention is placed on speed to the clinic. In late phase trials, there is an increased emphasis on accumulating process and product knowledge, reducing assay costs and increasing assay speed and efficiency.
The incredible success of genetically modified T cells in treating various hematological malignancies has accelerated the timeline to registration for cell therapies. To keep pace, process and analytical development are challenged to find solutions for the many commercial hurdles. As depicted in Figure 3, the focus during early development is usually on speed to the clinic. Appropriately, product safety is prioritized over commercial feasibility. A Phase 1 manufacturing process, for example, can be somewhat variable, inefficient, labor-intensive and costly. These characteristics are also true for many Phase 1 assays. As a product progresses toward registration, there will be an increased emphasis on accumulating process and product knowledge. The objective of this work is to develop a commercially viable process and assays. For the assay team, this involves creating an improved set of analytical tools, identifying the most meaningful methods, demonstrating the comparability of process changes, and increasing assay quality and efficiency. The autologous nature of many fast-to-market cell therapies is an added challenge. Since these products are manufactured on a patient-by-patient basis and are often destined for very sick patients, analytical testing can quickly become a bottleneck. To accommodate these challenges, it is necessary to invest in more rapid and high throughput analytical methods. The development of commercial manufacturing processes is similarly hindered by the lack of reliable potency assays, a universal challenge in the cell therapy field.
Potency Assay Development
The challenges related to the selection of cell therapy potency assays have been well documented [7]. Regulators require that a potency assay measures a relevant biological function of the active ingredient that is linked to the product’s clinical MOA. A potency assay is an important part of the release and stability program and, as such, will be used to determine the product’s specifications. To fit this purpose, the assay should be sensitive to changes in the product, produce quantitative data and be convenient for lot release testing. These last requirements underscore the need for assay validation. The field has struggled to select meaningful potency measurements, in part because the MOA of many cell therapies are varied, multifaceted and in some cases poorly defined. To handle this complexity, regulators have suggested that a single assay may not be sufficient and that a matrix of assays might be required to achieve a complete picture of a cell product’s potency [8]. The use of assays that are sensitive to changes in the product is a key to doing effective process development. These assays should measure the functional capability of the cells and not just physical and biological attributes. Applying functional tests when doing process development will provide valuable information about both the product and an assay’s potential as a potency measurement. Relevant functional tests should be considered in early development and judged based on their ability to meet the regulatory and practical requirements. The most likely potency measurements should be gauged for their ability to be validated. This is important since the development of a final potency assay may require iteration, refinement and a large investment in time to generate the necessary reagents (i.e., reference material) and to complete validation. The recommendations outlined above for the validation of methods, if started early in development, will be useful to deliver a high-quality, meaningful potency assay. The final and most challenging element of potency testing is the selection of specifications. Ideally, a link should be made between product efficacy (clinical response) and product characteristics. This can only be achieved through the close coordination of product characterization and clinical translation activities. It has yet to be determined whether this approach will be successful, and in the end, it may be sufficient to set potency specifications based on process capability or experience in the clinic.
Assay Scale-Up
Requirements around an assay’s throughput can vary depending on whether the product is allogeneic or autologous. In contrast to manufacturing for an allogeneic product, where a single production lot can potentially supply hundreds of people, autologous therapies require a new lot of cells to be made for every patient. Most cell therapy assays are novel, technically complex, time and labor intensive. Patient-specific manufacturing amplifies these problems since it requires assays to be executed more frequently. This is a significant driver for reducing assay speed, labor and cost, and increasing sample throughput. There are many ways to accomplish these tasks. Simplification of the assay processes should be the first approach. This may involve the elimination of lengthy or unnecessary steps, switching to antibody or reagent master mixes, creating data analysis templates and adopting electronic data recording tools. The method’s capacity to adapt to these modifications should be considered early in assay development. This is very important because large changes can influence the choice of assay platforms and require lengthy development times. Simplification, however, will pay dividends during method validation and routine use since it reduces the opportunities for operator error.
When considering ways to increase sample throughput, plans should encompass both in-process and release measurements. The batching of samples is a convenience that contract testing labs frequently use when running plate-based methods. Ideally, an assay’s sample throughput should be sufficient to take advantage of overlapping test days from parallel manufacturing runs. Many flow cytometry instruments are available with plate- or carousel-based acquisition devices and are compatible with software that is capable of performing a batch analysis of data. As an added advantage, plate-based assays will make it easier to increase the number of sample replicates, thereby enhancing measurement confidence. When developing these tests, special care should be taken to eliminate run order effects and fluorescent spill-over from adjacent wells. Controls should also be put in place to segregate samples and maintain chain of custody.
Automation is another option for improving throughput, as it offers the possibility of increasing assay speed and quality while reducing errors, labor and assay costs. Currently, there are no off-the-shelf automation solutions for cell therapy methods. A fully automated assay may be alluring because it significantly reduces the demand on labor and can facilitate transfer of a method to different QC labs. However, it may make sense to only automate the portions of an assay that create significant bottlenecks. For this reason, and because automation is a time consuming and costly investment, a modular approach may be preferred. Independent automation solutions may be considered for the sample preparation, instrument and data analysis components of an assay. This tactic may be more pragmatic, for many reasons. Modular automation is more accessible, easier to implement and can be applied across different analytical methods. Regardless of the approach, an assay should be simplified and the sources of error must be well understood before attempting to automate. Otherwise, it may prove difficult to bridge from the standard manual method.
Rapid Safety Tests
Cell therapy products are challenged by having lengthy manufacturing processes and in some cases extremely short shelf lives (e.g., days). Additionally, most of the field is targeting serious unmet medical needs, which are frequently accompanied by very sick patients. For these reasons, quickly completing the manufacturing and release process has become a top priority. Methods of shortening the cell production time are an active area of research. Analytical teams, meanwhile, are focused on ways to expedite release testing. Since cell therapy manufacturing relies on aseptic processing, sterility and mycoplasma tests are usually the rate-limiting steps in product release. The standard methods used for sterility (14 days) and mycoplasma (28 days) detection are lengthy, largely because they rely on the growth of organisms. When running these assays, careful engineering controls must be in place at the testing lab to guard against false positives. For this reason, most manufacturers choose to perform these assays at contract labs, who are experienced with the risks associated with running them. This further adds to assay turnaround time. When justified and accompanied by an appropriate control strategy, regulatory authorities have been amenable to infusing products before all release testing is complete. This approach, however, is not likely to work for a commercial product. To accommodate these challenges, cell therapy companies have been quick to adopt rapid and sensitive alternatives to the standard tests. These include bioluminescence and carbon dioxide-based substitutes for sterility and nucleic acid-based alternatives for mycoplasma testing. Regulatory guidance requires that these methods be validated and demonstrate comparability or superiority to the standard tests. Acceptance of the rapid methods by regulators, however, is still on a case-by-case basis. This is in part due to the years of historical precedent using the standard tests. Newly formed committees, which are bringing together cell therapy developers, standards organizations and regulators, promise to more clearly define the validation of these methods and how they can be used in manufacturing.
Conclusion
The recent clinical success of several cell therapies has made it an exciting time to work in the field. The unprecedented efficacy seen in these products has increased competition and expedited their path to registration. To realize the promise of these therapies, a large emphasis will be placed on analytical development to help meet regulatory needs and create robust and practical manufacturing processes. The use of a broad set of analytical tools in process development and manufacturing are keys to achieving these goals. When translating assays to the QC lab, it is necessary to understand the unique requirements of a quality test and how to effectively validate it. The complexity of cell therapy assays accentuates the need to invest properly in analytical development. By emphasizing careful planning, applying meaningful analytical measurements and through rigorous assay characterization, manufacturers will be well positioned to deliver on the commercial success of these promising treatments.
Financial & competing interests disclosure
The author has no relevant financial involvement with an organization or entity with a financial interest in or financial conflict with the subject matter or materials discussed in the manuscript. This includes employment, consultancies, honoraria, stock options or ownership, expert testimony, grants or patents received or pending, or royalties. No writing assistance was utilized in the production of this manuscript.
References
1. Heathman TRJ, Nienow AW, McCall MJ, Coopman K, Kara B, Hewitt CJ. Cell Therapy Manufacturing and Quality Control: Current Process and Regulatory Challenges. Regen. Med. 2015; 10(1): 49–64.
CrossRef
2. Wang X, Rivière I. Clinical manufacturing of CAR T cells: foundation of a promising therapy. Mol. Ther. Oncolytics 3 2016; 16015.
CrossRef
3. Vogt FG, Kord AS. Development of quality-by-design analytical methods. J. Pharm. Sci. 2011; 100(3): 797–812.
CrossRef
4. Pharmaceutical Development: ICH Q8/Q(8)R.
5. Lee JW, Devanarayan V, Barrett YC et al. Fit-for-purpose method development and validation for successful biomarker measurement. Pharm Res. 2006; 23(2): 312–28.
CrossRef
6. Cobb GW. Introduction to Design and Analysis of Experiments Edition 1, Wiley (1998).
7. Bravery C, Carmen J, Fong T et al. Potency assay development for cellular therapy products: an ISCT review of the requirements and experiences in the industry. Cytotherapy 2013; 15(1): 9–19.
CrossRef
8. Guidance for Industry Potency Tests for Cellular and Gene Therapy Products. US Department of Health and Human Services Food and Drug Administration Center for Biologics Evaluation and Research January 2011.
Affiliations
Chris Wiwi
Analytical Research and Development, Celgene, NJ, USA
This work is licensed under a Creative Commons Attribution- NonCommercial – NoDerivatives 4.0 International License.