Cord blood transplantation in pediatric inherited metabolic diseases: an update on clinical developments
Cell Gene Therapy Insights 2017; 3(7), 531-539.
10.18609/cgti.2017.046
Inherited metabolic diseases (IMDs), arising from genetic defects, including deficiencies in the production of the lysosomal enzymes (lysosomal storage disease [LSDs]) and abnormalities of peroxisomal function, are associated with devastating systemic processes and premature death. Allogeneic hematopoietic cell transplantation (HCT) has been shown to be a treatment option for a selected group of patients with IMD. Cord blood has proven to be the preferred cell source for HCT for a variety of reasons; most importantly due to the superior long-term outcomes associated with cord blood. In this article, the evolution of the use of cord blood as a cell source for HCT and the potential of this source as an adjuvant cellular therapy (e.g., targeting specific organs or cell types) to potentially reduce or completely prevent residual disease burden after HCT is reviewed.
Inherited metabolic diseases (IMDs) are a diverse group of diseases arising from genetic defects, including deficiencies in the production of the lysosomal enzymes (lysosomal storage disease [LSDs]) and abnormalities of peroxisomal function. These diseases are characterized by devastating systemic processes that may affect bone integrity, growth and development, cardiopulmonary status, the airway, hearing and vision, neurologic and cognitive function, resulting in early death. Allogeneic hematopoietic cell transplantation (HCT) has been shown to be a treatment option for a selected group of patients with an IMD. Timely diagnosis and immediate referral to an ‘IMD specialist’, with discussion on these patients in a multidisciplinary team including a transplant physician, are essential steps. Cord blood (CB) has proven to be the preferred cell source for HCT for a variety of reasons: for example, prompt availability (in sometimes rapidly progressive disease), lower probability of HCT-related complications such as graft-versus-host disease (GvHD), and higher enzyme levels after HCT. Survival rates with donor cell engraftment are nowadays over 95% in specialized centers using harmonized individualized treatment protocols; for example, conditioning regimens and GvHD-prophylaxis. Although HCT has proven to be a safe procedure and results in long-term survival, it is not a complete cure for most of these patients. Most patients will face a significant disease burden, which remains a clear medical need. Earlier transplantation and achieving higher enzyme levels after HCT may help to reduce this burden. In this article, the evolution of the use of cord blood as a cell source for HCT is reviewed as well as the potential of this source as an adjuvant cell therapy to potentially reduce or completely prevent residual disease burden after HCT.
Rationale for allogeneic HCT in IMD
The demonstration of the principle of ‘cross correction’ established that the accumulation of substrate in the cells of patients with lysosomal enzyme deficiencies could be reduced in the presence of cells producing the missing enzyme [1]. With this as a rationale, allogeneic HCT was explored in numerous lysosomal diseases, including Mucopolysaccharidosis type-1, Hurler phenotype (MPS-1H), other MPS diseases and in the inherited leukodystrophies; metachromatic leukodystrophy (MLD), X-linked adrenoleukodystrophy (X-ALD) and globoid cell leukodystrophy (GLD; or Krabbe’s disease) [2]. Donor cell engraftment subsequently results in donor microglia cell reconstitution in the brain of the patients, which result in enzyme delivery in the brain, resulting in (partial) correction of the CNS disease [3-5]. HCT has since become the ‘standard-of-care’ in a selected group of disorders (e.g., MPS-1H, MLD and early X-ALD) based on a variety of early and late outcome studies, including comparison studies with natural course of the disease [6-8]. Since the first HCT in an MPS-1H patient [9], over 2500 patients with IMD have been transplanted worldwide [2]. Treatment recommendations may vary significantly, even within a particular diagnosis. These decisions reflect the type of disorder, its predicted phenotype (which may sometimes be difficult, in particular for X-ALD patients where no genotype–phenotype correlations exist, the extent of disease progression, family values and expectations and the risks and benefits associated with available therapies. HCT for an IMD is performed using donor-derived hematopoietic stem cells obtained from bone marrow (BM), growth factor-mobilized peripheral blood (PB) and in the last decade predominantly from umbilical cord blood (CB). Not only are outcomes improved because of better HLA matching of the cord blood units (due to larger inventory) but also higher cell doses of the cord blood units collected. Furthermore, improved and harmonized, individualized preparative regimens and supportive care has resulted in safer HCT. At this point, for patients with a non-malignant disease such as IMD, transplant related complications such as infections and GvHD, are relatively low, especially chronic-GvHD [6,10] and engrafted survival chances are over 90% in specialized centers.
Diseases that may benefit from transplantation
Although HCT has proven to influence the natural course of a variety of IEM significantly, not all diseases of phenotypes profit from HCT. The currently accepted IEM diagnoses are mucopolysaccharidosis (MPS) type 1 and the leukodystrophies described above. These diseases account for over 80% of the HCTs for IMD. The remaining which may profit from HCT are some very rare miscellaneous indications and are recently reviewed [2]. This review also verifies the place of HCT (or alternative treatments) for each disease, including the diseases discussed here. All transplant decisions are based on a balance of risk and potential benefits. There is a greater opportunity for benefit in patients with a milder phenotype and/or in patients who are earlier in the course of their disease [6,11,12]. Much of the morbidity (such as skeletal disease, neurological disease) associated with the disease itself is not reversed by HCT, except organomegaly in the IMDs. Treatment of the central nervous system (CNS) with transplant is primarily mediated by the engraftment of donor-derived microglial cells in the brain. As it takes several months to replace sufficient microglia cells with those derived by the donor, there is a delay in any significant benefits of transplant to the CNS [5]. Diseases affecting the brain that are rapidly progressive are therefore more difficult to treat with standard HCT (e.g., infantile GLD and MLD and advanced cerebral ALD). With improving results of HCT and reducing risks it is appropriate to consider whether diseases of milder phenotype might be considered for HCT, as the alternative is weekly 4–6 hour infusions of enzyme. This might include milder IMD currently managed with enzyme replacement therapy (ERT), such as Hurler-Scheie, attenuated MPSII or MPSVI.
Furthermore, much of the older literature describing outcomes with HCT in any particular disease is out-dated: e.g. it is said that HCT is ineffective in MPSII (Hunter), but such a statement is derived from HCT experience in ‘symptomatic patients’ using sibling (usually carrier) donor grafts, historically associated with high frequency on mixed-chimerism [2,10]. The outcomes may be different in those same patients if it had been performed earlier (‘pre-symptomatic’ status) and using an unrelated cord blood donor, where enzyme delivery would have been optimized as shown in some recent studies [6,10–12]. Thus, some of these HCT questions must be re-evaluated in this modern era. Collaboration between centers for these re-evaluations is of utmost importance since these diseases are rare and sometimes even ultra-rare.
Evolution of the medical need & strategies to optimize the outcomes
Until a decade ago, the enthusiasm for transplanting patients with an IMD was hampered by high rates of graft-failure and transplantation-related mortality resulting in relatively low engrafted survival rates of approximately 50% [13,14]. From the mid-2000s larger international collaborative studies identified predictors for graft-failure associated with poor engrafted-survival rates: T-cell depleted grafts and reduced intensity conditioning. Busulfan (BU) with therapeutic drug monitoring (targeting to a myelo-ablative exposure) appeared to be a predictor for higher event-free survival (survival with donor cell engraftment) in comparison to prior experiences [10,13,15]. This data has led to international guidelines [16]. These guidelines included a standardized BU/cyclophosphamide (CY) conditioning regimen and the use of CB as a preferred graft-source, second only to non-carrier matched sibling BM. This transplant protocol with well-matched grafts resulted in a significant improved ‘engrafted survival’ rates of over 90% in larger experienced HCT-centers. Recently, the conditioning was modified to Busulfan targeted to myeloablative exposure (cumulative 90 mg*h/L + Fludarabine 160 mg/m2) (Box 1). Conditioning comparisons (BuCy vs FluBu) showed similar HCT outcomes but with reduced toxicity [17,18]. To further improve the safety of HCT, it was shown that individualizing anti-thymocyte globuline results in better predictable immune-reconstitution associated with lower infection-rates and TRM [19,20].
Over the past decade, unrelated CB has been used with increasing frequency as a graft source for HCT in children with IMD. CB offers several advantages over BM or PB, including reduced time to HCT, greater tolerance for HLA-mismatch, lower incidence and severity of GvHD in particular chronic-GvHD, reduced likelihood of transmitting viral infections and rapid T-cell immune reconstitution provided using individualized anti-thymocyte globuline [20]. Recent collaborative studies also suggest that the highest ‘engrafted survival’ rates are achieved in patients receiving an identical matched sibling donor or an identical (6/6) unrelated CB, followed by 5/6 matched CB or 10/10 matched unrelated donor [10]. Interestingly, in this analyses almost all CB recipients had full-donor chimerism associated with ‘normal-enzyme’ levels, while mixed-chimerism was more frequently seen in matched sibling and volunteer unrelated adult donors (up to 30–50% of the transplanted patients). It is also important to recognize that most matched sibling donors are carriers, influencing post-transplant enzyme levels. Enzyme levels after HCT appears to be essential as lower enzyme levels are associated with more compromised long-term outcomes [16,21]. Aldenhoven et al. recently analyzed these late outcomes in a large international long-term outcome study: patients with enzymes below the lower limit of normal had more serious complications such as cord compression surgery and nocturnal respiratory support compared to patients with normal enzymes [6]. Additionally, growth was significantly better in patients with normal enzyme levels. An interesting and important observation from this study was that the enzymes levels achieved after CB transplantation (with full-donor chimerism), compared to full-donor chimerism in unrelated donor setting, achieved significantly higher enzymes levels at a median follow up of 9 years (Figure 1), which has a potential health advantage for these patients on the longer term [6]. These observations further support the use of cord blood as cell source over bone marrow from either related or unrelated donors.
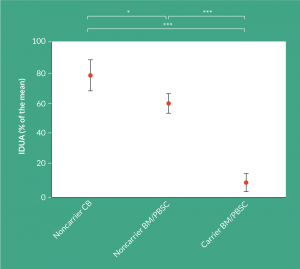
Figure 1: Obtained IDUA levels post-HCT subdivided by donor source. IDUA level, expressed as % of the mean, subdivided by the donor source used (noncarrier CB; n = 69, noncarrier BM/PBSC; n = 54, carrier BM/PBSC; n = 19). Only patients receiving a donor with a known carrier status and achieving full donor chimerism (>95%) were included in this analysis. 64% of the noncarrier CB group were transplanted in a center where donor selection was in part based on highest IDUA
enzyme level. Shown are the mean, with corresponding 95% confidence intervals. BM:
Bone marrow; CB: Cord blood; HCT: Hematopoietic cell transplantation; IDUA: Alpha-L-iduronidase; PBSC: Peripheral blood stem cells. *P<0.05; ***P<0.001.
One of the needs for the upcoming years is development of protocols that can further reduce toxicity with equivalent engraftment rates. Especially when this will give benefits in regards to fertility and more normal endocrine function after HCT. Agents such as treosulfan are considered to be less toxic, there is however data that clearly suggests that busulfan may provide superior microglial engraftment (essential for earlier and higher delivery of the missing enzyme in the brain) [5]. Another strategy to enhance safety of the transplant is also suggested to shorten the neutropenic period by using expanded CB grafts. A trial was planned to open last year (NCT02715505) to study the safety of a CB-expanded graft. The supposed advantage of this strategy is selecting a better-matched graft (which is associated with less GvHD) and a shorter neutropenic period (associated with less infections).
Another need is the residual disease burden. For this the enzyme dose delivered by HCT appeared to be important in patient’s late outcomes. Thus strategies increasing the enzyme delivery may further improve the outcomes. Several lines of evidence suggest that autologous cells that are genetically modified to express the deficient enzyme may further improve outcome compared to wild type donors [22,23]. Furthermore, gene therapy has the capacity to enhance enzyme secretion not only by increasing the gene copy number but also by re-regulating gene expression so that it occurs in monocytic and other mature blood cells. As the enzyme level has been shown to be a predictor for long-term outcomes (e.g., skeletal disease) higher enzymes achieved with gene therapy may positively influence the long-term outcomes in the future. Biffi and colleagues have demonstrated clinical benefit to such an approach in infantile MLD [23]. Whether gene therapy is superior to HCT, and effective in all IMD, needs to be determined after longer-term follow up and comparison with standard HCT, including CB transplantation.
Alternatively, adjuvant cell therapy options may reduce or prevent residual disease burden. As CB has proven to be safe and effective in treating patients with IMD, adjuvant cell therapies are currently being developed to achieve better correction of tissues that are not optimally corrected after conventional CB transplantation or to bridge the engraftment of donor microglia cells in the brain. An example is DUOC-01 cells (oligodendrocyte-like cells) [24], a cell therapy product derived from the same unrelated CB as used for transplantation. In a currently open clinical trial (NCT02254863), patients with an IMD receive intrathecal administration of these cells, aiming for earlier enzyme replacement in the brain, especially for patients with rapidly progressive cerebral disease.
Translational insight
Because the use of CB in IMD patients is associated with remarkably high engrafted-survival rates, this hematopoietic cell source has been used increasingly. Moreover, recipients of unrelated CB donors more often achieve full donor chimerism and subsequently normal enzyme levels, and UCB units are readily available reducing the age at HCT. Because a normalized enzyme level after HCT, lower age at time of transplant and disease status at HCT are highly important predictors for superior long-term prognosis of IMD patients, UCB grafts are considered as one of the most attractive sources for HCT in these patients.
Although short-term transplant-related outcomes of unrelated CB transplantation are favorable, especially in patients treated early in the course of their disease, in most diseases where residual disease burden and disease progression is not fully halted or slowed down over time, there remains an urgent medical attention. It is likely that augmented and combination therapies, such as gene or augmentative cellular therapies, probably will be needed to provide reduction of residual disease burden and optimal quality of life for these patients after transplantation. In addition, newborn screening will also allow for the option of unrelated CB transplantation to be offered to affected patients at an earlier state of their disease.
financial & competing interests disclosure
The author has no relevant financial involvement with an organization or entity with a financial interest in or financial conflict with the subject matter or materials discussed in the manuscript. This includes employment, consultancies, honoraria, stock options or ownership, expert testimony, grants or patents received or pending, or royalties. No writing assistance was utilized in the production of this manuscript.
References
1. Fratantoni JC, Hall CW, Neufeld EF. Hurler and Hunter syndromes: mutual correction of the defect in cultured fibroblasts. Science 1968; 162(3853): 570–2.
CrossRef
2. Boelens JJ, Orchard PJ, Wynn RF. Transplantation in inborn errors of metabolism: current considerations and future perspectives. Br. J. Haematol. 2014; 167(3): 293–303.
CrossRef
3. Neumann H. Microglia: a cellular vehicle for CNS gene therapy. J. Clin. Invest. 2006; 116(11): 2857–60.
CrossRef
4. Biffi A, Capotondo A, Fasano S et al. Gene therapy of metachromatic leukodystrophy reverses neurological damage and deficits in mice. J. Clin. Invest. 2006; 116(11): 3070–82.
CrossRef
5. Capotondo A, Milazzo R, Politi LS et al. Brain conditioning is instrumental for successful microglia reconstitution following hematopoietic stem cell transplantation. Proc. Natl Acad. Sci. USA 2012; 109(37): 15018–23.
CrossRef
6. Aldenhoven M, Wynn RF, Orchard PJ et al. Long-term outcome of Hurler syndrome patients after hematopoietic cell transplantation: an international multicenter study. Blood 2015; 125(13): 2164–72.CrossRef
7. van Rappard MD DF. Efficacy of HCT in MLD. Blood 2016; 127(24): 3094–8.
Webiste
8. Escolar ML, Poe MD, Provenzale JM et al. Transplantation of umbilical-cord blood in babies with infantile Krabbe’s disease. N. Engl. J. Med. 2005; 352(20): 2069–81.
CrossRef
9. Hobbs JR, Hugh-Jones K, Barrett AJ et al. Reversal of clinical features of Hurler’s disease and biochemical improvement after treatment by bone-marrow transplantation. Lancet 1981; 2(8249): 709–12.
CrossRef
10. Boelens JJ, Aldenhoven M, Purtill D et al. Outcomes of transplantation using various hematopoietic cell sources in children with Hurler syndrome after myeloablative conditioning. Blood 2013; 121(19): 3981–7.
CrossRef
11. Staba SL, Escolar ML, Poe M et al. Cord-blood transplants from unrelated donors in patients with Hurler’s syndrome. N. Engl. J. Med. 2004; 350(19): 1960–9.
CrossRef
12. Miller WP, Rothman SM, Nascene D et al. Outcomes after allogeneic hematopoietic cell transplantation for childhood cerebral adrenoleukodystrophy: the largest single-institution cohort report. Blood 2011; 118(7): 1971–8.
CrossRef
13. Boelens JJ, Wynn RF, O’Meara A et al. Outcomes of hematopoietic stem cell transplantation for Hurler’s syndrome in Europe: a risk factor analysis for graft failure. Bone Marrow Transplant. 2007; 40(3): 225–33.
CrossRef
14. Peters C, Balthazor M, Shapiro EG et al. Outcome of unrelated donor bone marrow transplantation in 40 children with Hurler syndrome. Blood 1996; 87(11): 4894–902.
Webiste
15. Boelens JJ, Bierings MB, Tilanus M, Lie J, Sedlacek P. Outcomes of transplantation of unrelated cord blood in children with malignant and non-malignant diseases: an Utrecht-Prague collaborative study. Bone Marrow Transplant. 2009; 43(8): 655–7.
CrossRef
16. Boelens JJ, Bierings M, Wynn RF. HSCT HandBook EBMT/ESH 2012: HSCT for children and adolescents. 2012; 1–14.
17. PhD IHB, PharmD AL, van Reij PharmD EML et al. Articles Association of busulfan exposure with survival and toxicity after haemopoietic cell transplantation in children and young adults: a multicentre, retrospective cohort analysis. Lancet Haematol. 2016; 1–11.
Webiste
18. Bartelink IH, van Reij EML, Gerhardt CE et al. Fludarabine and exposure-targeted busulfan compares favorably with busulfan/cyclophosphamide-based regimens in pediatric hematopoietic cell transplantation: maintaining efficacy with less toxicity. Biol. Blood Marrow Transplant. 2014; 20(3): 345–53.
CrossRef
19. MD RA, van Kesteren PhD C, Zijde BSc der CMJ-V et al. Articles Association between anti-thymocyte globulin exposure and CD4+ immune reconstitution in paediatric haemopoietic cell transplantation: a multicentre, retrospective pharmacodynamic cohort analysis. Lancet Haematol. 2015; 2(5): 1–10.
DOI
20. Admiraal R, Lindemans CA, van Kesteren C et al. Excellent T-cell reconstitution and survival depend on low ATG exposure after pediatric cord blood transplantation. Blood 2016; 128(23): 2734–41.
CrossRef
21. Aldenhoven M, Boelens JJ, de Koning TJ. The clinical outcome of Hurler syndrome after stem cell transplantation. Biol. Blood Marrow Transplant. 2008; 14(5): 485–98.
CrossRef
22. Biffi A, Montini E, Lorioli L et al. Lentiviral hematopoietic stem cell gene therapy benefits metachromatic leukodystrophy. Science 2013; 341(6148): 1233158.
CrossRef
23. MD MS, MD LL, MD FF et al. Articles Lentiviral haemopoietic stem-cell gene therapy in early-onset metachromatic leukodystrophy: an ad-hoc analysis of a non-randomised, open-label, phase 1/2 trial. Lancet 2016; 1–12.
DOI
24. Kurtzberg J, Buntz S, Gentry T et al. Preclinical characterization of DUOC-01, a cell therapy product derived from banked umbilical cord blood for use as an adjuvant to umbilical cord blood transplantation for treatment of inherited metabolic diseases. Cytotherapy 2015; 17(6): 803–15.
CrossRef
Affiliations
Jaap Jan Boelens1,2
1 University Medical Center Utrecht, Pediatric Blood and Marrow Transplantation Program, Utrecht, The Netherlands
2 Laboratory Translation Immunology, University Medical Center Utrecht, Utrecht, The Netherlands

This work is licensed under a Creative Commons Attribution- NonCommercial – NoDerivatives 4.0 International License.