Cell and gene therapies: improving and extending quality of life for seniors
Cell Gene Therapy Insights 2015; 1(1), 5-13
10.18609/cgti.2015.002
Editorial
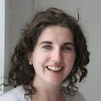
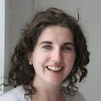
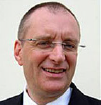
Cell and gene therapy represents a new paradigm in human healthcare with the ability to resolve unmet medical needs by directly targeting the underlying cause of disease. One of the major social drivers in the 21st century is to address the medical needs of the aging population. Today in the USA, UK, and Japan, people over the age of 65 make up 14%, 18% and 26% of the population, respectively [1]. However, over the coming decades these proportions will dramatically rise due to a combination of longer life spans and aging baby boomers. With increasing age comes increasing health challenges. Biological aging reduces the ability of organs, tissues and cells to respond effectively to everyday wear-and-tear, trauma, disease and cancer. “Once-and-done” cell and gene therapies with their potential to transform patients’ lives (and, in some cases, even provide a cure) are a major step-change from the traditional pharmaceutical “a-pill-a-day-for-life” approach, which at best only manages disease and related symptoms. So what do cell and gene therapies potentially offer seniors to enable a sustained quality of life? This editorial highlights cell and gene therapies that specifically target medical conditions associated with aging. Below are examples of significant causes of morbidity and mortality in seniors where cell and/or gene therapies are either already available in the clinic or in the clinical trials’ phase of development.
Cardiovascular disease
Heart disease is the leading cause of death in the USA. On the basis of 2011 mortality data, over 2,150 Americans died of cardiovascular disease (CVD) each day, an average of 1 death every 40 seconds. The vast majority (over 600,000) who died of CVD in 2011 were over 65 [2]. Around half of those who develop heart failure die within 5 years of diagnosis [3]. Some of the most advanced clinical trials in cell therapy are in CVD, for example the pharmaceutical company Teva Pharmaceutical Industries (Petach Tikva, Israel), together with Mesoblast (Melbourne, Australia) are currently conducting a large Phase 3 (pivotal study) in heart failure patients [4]. This Phase 3 trial builds on a promising Phase 2 trial led by Emerson Perrin (Texas Heart Institute, Houston, TX, USA) that suggested high-dose allogeneic mesenchymal precurser cells “…may decrease major clinical events associated with progressive heart failure for at least 3 years, including repeated hospitalizations or death” [5,6]. There are many other ongoing CVD studies using cell and gene therapies in heart attack (myocardial infarction), heart failure and peripheral artery disease (PAD)/critical leg ischemia. Ongoing gene therapy trials include the Phase 3 ASPIRE angina trial led by Taxus Cardium (San Diego, CA, USA) examining the potential of delivering to the heart, via an adenovector, fibroblast growth factor-4 (FGF-4) gene [7]. FGF-4 activates a number of angiogenesis pathways, including the vascular endothelial growth factor (VEGF) pathway, thus promoting microvascular growth. Big Pharma is already involved in CVD cell therapy including Celgene (Summit, NJ, USA) who are running a Phase 1 trial for PAD, and Johnson & Johnson (New Brunswick, NJ, USA) who have a strategic partnership with Capricor Therapeutics (Beverley Hills, CA, USA) and are now in Phase 2 for the prevention of heart failure through the deployment of donor cardiospheres to promote myocardial regeneration [8,9].
‘Once-and-done’ cell and gene therapies…are a major step-change from the traditional pharmaceutical ‘a-pill-a-day-for-life’ approach.
Cancer
Advancing age is a high risk factor for cancer, with persons over 65 accounting for 60% of newly diagnosed malignancies and 70% of all cancer deaths [10]. The incidence of cancer in those over 65 is 10 times greater than in those younger than 65 and the cancer death rate is 16 times greater in patients over 65 compared to younger patients. More than 70% of the mortality associated with many cancers including prostate, bladder, bowel, lung, uterus and pancreas occur in patients 65 and older [10]. Cell and gene therapies are starting to dramatically address cancer by boosting the patient’s own immune system to eradicate malignant cells, including metastases. Transformative scientific and clinical breakthroughs are now being made by employing cell- and gene-based therapies to combat various forms of cancer. Cell-based immunotherapy is one of the hottest areas in science and translational medicine [11]. The four major cancers of persons over 65 – bowel, breast, lung and prostate – are all being targeted using either cell and/or gene therapy-based approaches to immunotherapy [12–15]. Other cancers associated with aging including hematological malignancies (e.g., chronic myeloid leukemia, non-Hodgkin’s lymphoma and malignant myeloma), mesothelioma, and head and neck cancers are also being pursued in the clinic [16–19].
[pullquote type=”right”]Cell and gene therapies are starting to dramatically address cancer by boosting the patient’s own immune system to eradicate malignant cells, including metastases.[/pullquote] The results to date from these clinical studies are highly encouraging, demonstrating in certain instances step-change outcomes (and, in some cases, cures) over conventional therapies. The first cell-based immunotherapy is expected to get FDA approval in 2016. The developer, Novartis (Basel, Switzerland) are in the final stages of submitting their Investigational New Drug (IND) application to FDA. The vast majority of Big Pharma is now either directly involved in cell-based immunotherapies or have formed a strategic partnership with a cell and/or gene therapy company including Celgene, GlaxoSmithKline (Brentford, London, UK), Novartis, and Pfizer (New York, NY, USA).
Neurological diseases
Each year in the USA approximately 800,000 people suffer a stroke [20]. Nearly 75% of strokes occur in persons over the age of 65. The risk of having a stroke more than doubles each decade after the age of 55 [21]. Early trials in stroke suggest that a meaningful degree of lost function may be restored using cell therapy e.g., ReNeuron (Guildford, UK) [22]. Cell therapy for Parkinson’s disease goes back decades. Initial trials in the 1980s and 1990s demonstrated benefits for a few patients but others only had side effects [23]. The principle challenges were the source and quality of the cells. Today these issues are now mainly resolved, thus enabling trials with high quality cells to progress, for example the multi-national TRANSEURO Parkinson’s disease study led by Roger Barker at Cambridge University (Cambridge, UK) [24]. The commonest cell types being considered as starting materials are pluripotent stem cells, both human embryonic and induced pluripotent stem (iPS) cells. One of the first clinical studies to use iPS cells to derive dopaminergic cells is likely to be in Japan. The trial led by Jun Takahashi at the Center for iPS Cell Research and Application (CiRA), Kyoto University (Kyoto, Japan) is planning to be ready to treat their first Parkinson’s patients in 2018 [25]. Industry is likewise active in the Parkinson’s disease cell therapy space.[pullquote type=”left”]The vast majority of Big Pharma are now either directly involved in cell-based immunotherapies or have formed a strategic
partnership with a cell and/or gene therapy company.[/pullquote] Recently, Sumitomo Dainippon Pharma (Osaka, Japan), CiRA and Hitachi (Tokyo, Japan) agreed a collaborative research program based on the use of iPS cells. Sponsored by the Japanese government, the plan is for CiRA and Sumitomo Dainippon Pharma to jointly develop the clinical aspects whilst in parallel, Hitachi produces the necessary automated cell culturing technology to enable efficient mass-production and a stable supply of safe and consistent quality cells [26].
Deafness
Around a third of 65 year olds have hearing impairment, a number that rises to 50% by age 75 [27]. Apart from lip and speech reading, the only available treatments are prosthetic devices, such as assisted-listening devices, hearing aids and cochlear implants. These devices can improve hearing, however, their adoption is low; amongst adults aged 70 and older with hearing loss who could benefit from hearing aids, less than 30% have ever used them [28]. Approximately 90% of hearing loss is caused by damage to sensory hair cells in the inner ear (sensorineural deafness). Damage to hair cells is irreparable and is due to multiple causes including aging, noise-induced trauma, and certain drugs that have ototoxic side effects, such as as the commonly prescribed antibiotic, gentamycin. Until the late 1980s it was common dogma that the process of hair cell regeneration was impossible in higher invertebrates, including humans. However, through reaserch on the embryology of the ear, a number of genes have been identified as potential candidates to deploy as gene therapies to regenerate lost hair cells [29]. The most promising candidate to date is Atonal (Atoh1), which is the primary gene responsible for hair cell differentiation. Atonal is switched off before birth, however, if switched back on again, it biologically triggers the regeneration of sensory cells, leading to the restoration of hearing in animal models [30]. In 2014, GenVec (Gaithersburg, MD, USA) in partnership with Novartis commenced a gene therapy clinical trial to test the concept in humans [31]. A recombinant adenovirus 5 vector containing the human Atonal transcription factor (Hath1) cDNA is administered via intra-labyrinthine infusion to patients with severe to profound bilateral hearing loss. The first patient was treated in October 2014, and the results of this Phase 1/2, 45-patient study are expected in 2017.
Blindness
Age-related macular degeneration (AMD) accounts for approximately 9% of all blindness worldwide and is the most common cause of blindness in developed countries, particularly in people older than 60 years [32]. There have been significant advances in the management of exudative (“wet”) AMD with the introduction of anti-angiogenesis therapy, including Lucentis® (ranibizumab) from Genentech (South San Francisco, CA, USA) and Eylea® (aflibercept) from Regeneron (Tarrytown, NY, USA) and Bayer (Leverkusen, North Rhine-Westphalia, Germany). However, the “dry” variant, which is far more common (prevalence 70–90%) in developed countries, remains an unmet medical need. It should also be noted that whilst the terms “wet” and “dry” are a good short-hand for the features of AMD, in reality the disease is generally thought to be a continuum involving both forms. It is estimated that nearly 2 million Americans aged 40 years and older are affected by AMD and an additional 7 million with large drusen (deposits under the retina that are associated with AMD) are at substantial risk of developing the disease. The number of people with AMD is estimated to reach 3 million in 202033. The disease impacts 1 in 4 people by the age of 80 years [34]. Cell and gene therapy clinical trials are now actively underway to prevent and/or reverse AMD by replacing the lost retinal pigment epithelial cells (RPEs). The rationale is that the cause of AMD is considered to be the degeneration of the RPEs that form a monolayer at the back of the eye, directly under the retina. The role of RPE cells includes maintaining the health and functionality of the rods and cones that make up the retina. When RPEs die, this leads to the rods and cones they support also dying, resulting in loss of vision. Early data using human embryonic stem cell-derived RPE cells by Ocata Therapeutics (Marlborough, MA, USA) potentially suggests that replacing RPEs may not only slow AMD, but possibly reverse blindness [35,36]. University College London (London, UK) in collaboration with Pfizer are expected to start a similar clinical trial in the near future, but instead of injecting loose cells in suspension under the retina, the team plan to use a 6 x 3 mm polyester patch to support a pre-formed confluent RPE monolayer that will be inserted immediately under the retina [37].
The first trial in man using iPS cells as a starting source for a cell therapy commenced in Japan in 2014. The study uses autologous iPS cells to derive RPEs. A Japanese lady in her 70s was the first patient to be treated by Masayo Takahashi and her team at the RIKEN Center for Developmental Biology (Kobe, Japan). During the processing of cells for the second patient, the autologous cells were found to contain a genetic mutation, a finding that contributed to a recent decicion to temporarily suspend the trial. In July 2015, Takahashi stated, “It is true that a mutation was found in the cells before transplantation into the second patient, and this is something that we took into account when we made the decision to suspend (for the time being) the study, but the main reason for the suspension was the regulatory change. The mutated genes were not driver genes for tumor formation and the finding of the mutation was not something that called for a halt of the trial according to the protocol. We are planning to move to allogeneic transplantation because we found in the animal experiments that the iPS-RPE cells cause little or no immune response if the HLA is matched” [38]. This allogeneic iPS-cell-based trial is now actively being taken forward by a joint venture between Healios (a RIKEN VENTURE company in Tokyo, Japan) and Dainippon Sumitomo Pharma (Osaka, Japan) [39,40]. Other companies with clinical trials for dry AMD include StemCell Inc (Newark, CA, USA) who are in a Phase 2 study using human neuronal stem cells [41].
Skin Ulcers
Chronic wounds, which include venous leg ulcers, diabetic foot ulcers, arterial insufficiency, and pressure ulcers, disproportionately afflict older adults and impose substantial morbidity and mortality on millions of older Americans [42]. The great majority of chronic wounds are associated with conditions more common in older people, including vascular disease, venous insufficiency, unrelieved pressure, or diabetes mellitus. In addition, a disproportionate and increasing number of older adults undergo surgery and are at risk for wound complications [42]. Decubitus ulcers (pressure sores) are one of the most common and often disabling conditions that affect frail older people and have been estimated to impact between 2 and 28% of nursing home residents [43]. For the ambulatory elderly, venous leg ulcers are the most common type of chronic wound. David Margolis (University of Pennsylvania, PA, USA) reported that in people between 65 and 95 years of age, the annual prevalence of venous leg ulcers was approximately 2% of the population [44]. To date over 1 million patients have received FDA-approved tissue-engineered products, Apligraf® and Dermagraft® (Organogenesis, MA, USA), to treat chronic leg ulcers. However, in general, the development of evidence-based clinical protocols for the therapeutic use of cellular and tissue-engineered approaches has been challenging due to a lack of well-controlled and comparative data, and clear mechanism(s) of action, further hampered by the need for better defined clear regulatory and reimbursement pathways [42]. However, clinical trials are progressing, for example a Phase 1 trial of adipose-derived stem cells to treat the more severe full-thickness skin loss decubitus ulcers (Stages III and IV) is starting at the Mayo Clinic (Jacksonville, Florida, USA) [45]. To support the field the US Wound Registry (USWR) was created in 2005 in response to the Medicare Coverage Advisory Committee meeting earlier in the year, “The Usual Care of Chronic Wounds”. Since 2005, the USWR has been collecting data to create a “Cellular and Tissue Based Therapy Registry (CTPR) for Wounds” [46]. The target is to make available US national data on outcomes and effectiveness of treatment for outpatients who have received cell and tissue-based products across the entire spectrum of chronic wounds.
Urinary incontinence
The number of older adults with urinary incontinence (UI) is increasing rapidly worldwide as the population ages [47]. In general the incidence of UI in nursing homes in the USA is high and according to a series of studies, it affects between 43 and 81% of residents [47]. For example, a recent study across all Medicare- or Medicaid-licensed nursing homes in Minnesota, USA found the prevalence to be approximately 66% [48]. UI is increasingly common with age and increases the risks of falls and fractures, urinary tract infections and pressure sores. A number of trials are underway, including some in late stage, using a variety of different cell types to treat female stress UI, for example, Cook Medical (Bloomington, IN, USA) are running an international, multicenter, randomized double blind Phase 3 pivotal study using autologous muscle-derived cells [49,50]. In general, the initial results from female UI studies appear highly encouraging with improved continence and quality of life [51]. However, it remains unclear what cell type, or combination of cell types, is required and whether there is a need for additional injectable biomaterials [51]. Male stress UI affects a substantial number of patients after undergoing prostatic surgery. The incidence for these patients has been reported to be as high as 87% and is a critical determinant in postoperative health-related quality of life [52]. A number of different approaches using stem cells have been explored, including using stem cells derived from bone marrow, adipose tissue, and skeletal muscle [52]. For example, a clinical trial in 63 patients looking at injecting stem cells into the rhabdospincter (external urethral sphincter) and fibroblasts into adjacent urethral submucosa following radical prostatectomy for prostate cancer has produced encouraging results, with 12 month follow-up data suggesting sphincter regeneration and improved quality of life [53]. Other cell therapy approaches for male UI following radical prostatectomy include a Cytori Therapeutics (San Diego, CA, USA) supported Japanese government-funded study based on a successful feasibility trial conducted by Nagoya University (Nagoya, Japan) [54].
Conclusion
[pullquote type=”left”]In 2015, we are at the beginning of the cell and gene therapy revolution that will forever change the way medical conditions are treated.[/pullquote]
In 2015, we are at the beginning of the cell and gene therapy revolution that will forever change the way medical conditions are treated. A major focus for the sector, academia and industry alike, are the medical conditions associated with old age and their transformation from the current “status quo” of being expected with aging and therefore something to have to live with, to prevention or cure. The above examples are of cell and gene therapy activities that are in the clinic today, however, these are just the tip of a fast growing iceberg, with many more potential therapies and enabling technologies, such as gene editing, underway in laboratories around the world. Within the next few decades, cell and gene therapies will help transform the lives of many older people by improving and extending their quality of life.

This work is licensed under a Creative Commons Attribution- NonCommercial – NoDerivatives 4.0 International License.
Affiliations
Chris Mason
Professor of Regenerative Medicine Bioprocessing, Advanced Centre for Biochemical Engineering, University College London, Bernard Katz Building, Gordon Street, London WC1H 0AH, UK
Emily Culme-Seymour
Director, London Regenerative Medicine Network, MedCity, 2 Royal College Street, London NW1 0NH, UK
Geoff MacKay
Partner, Proteus Venture
Senior Partner, Proteus Venture Partners LLC, 8 Redberry Ridge, Portola Valley, CA 94028, USA
Financial & Competing Interests Disclosure
The authors have no relevant financial involvement with an organisation or entity with a financial interest in or financial conflict with the subject matter or materials discussed in the manuscript. This includes employment, consultancies, honoraria, stock options or ownership, expert testimony, grants or patents received or pending, or royalties.
No writing assistance was utilised in the production of this manuscript.
References
1. The World Bank www.data.worldbank.org/indicator/SP.POP.65UP.TO.ZS
2. Mozaffarian D, Benjamin EJ, Go AS et al Heart disease and stroke statistics–2015 update: a report from the american heart association. Circulation 2014; 131(4): e29–e322.
3. Centers for Disease Control and Prevention (CDC) http://www.cdc.gov/dhdsp/data_statistics/fact_sheets/fs_heart_failure.htm
4. www.clinicaltrials.gov/show/NCT02032004
5. Perin EC, Borow KM, Silva GV et al. A Phase II Dose-Escalation Study of Allogeneic Mesenchymal Precursor Cells in Patients With Ischemic or Non-Ischemic Heart Failure. Circ. Res. 6 Jul 2015 Epub ahead of print.
CrossRef
6. http://ir.mesoblast.com/DownloadFile.axd?file=/Report/ComNews/20150715/01641407.pdf
7. www.clinicaltrials.gov/show/NCT01550614
8. www.clinicaltrials.gov/show/NCT02460081
9. www.clinicaltrials.gov/show/NCT01458405
10. Berger NA, Savvides P, Koroukian SM et al. Cancer in the elderly. Trans. Am. Clin. Climatol. Assoc. 2006; 117: 147–56.
11. Couzin-Frankel J. Breakthrough of the year 2013. Cancer Immunotherapy. Science 2013; 342(6165): 1432–3.
CrossRef
12. www.clinicaltrials.gov/show/NCT02111850
13. www.clinicaltrials.gov/show/NCT01837602
14. www.adaptimmune.com/wp-content/uploads/2015/07/ADAP-MAGE-A10-IND-acceptance-release-FINAL-2-July-2015.pdf
15. www.clinicaltrials.gov/show/NCT00065442
16. Rapoport AP, Stadtmauer EA, Binder-Scholl GK et al. NY-ESO-1-specific TCR-engineered T cells mediate sustained antigen-specific antitumor effects in myeloma. Nat. Med. 2015; 21(8): 914–21.
CrossRef
17. Gill S, June CH. Going viral: chimeric antigen receptor T-cell therapy for hematological malignancies. Immunol. Rev. 2014; 263(1): 68–89.
CrossRef
18. Beatty GL, Haas AR, Maus MV. Mesothelin-specific chimeric antigen receptor mRNA-engineered T cells induce anti-tumor activity in solid malignancies. Cancer Immunol. Res. 2014; 2(2): 112–20.
CrossRef
19. Papa S, van Schalkwyk M, Maher J. Clinical Evaluation of ErbB-Targeted CAR T-Cells, Following Intracavity Delivery in Patients with ErbB-Expressing Solid Tumors. Methods Mol. Biol. 2015; 1317: 365–82.
CrossRef
20. Centers for Disease Control and Prevention (CDC): www.cdc.gov/stroke/docs/consumered_stroke.pdf
21. Mullner RM. Encyclopedia of Health Services Research. Volumes 1 and 2, Chapter ‘Mortality, Major Causes in the United States’, Sage Publications, Inc., 2009; p785.
22. www.reneuron.com/long-term-clinical-data-from-pisces-stroke-trial/
23. Kefalopoulou Z, Politis M, Piccini P et al. Long-term clinical outcome of fetal cell transplantation for Parkinson disease: two case reports. JAMA Neurol. 2014; 71(1): 83–7.
CrossRef
24. www.clinicaltrials.gov/show/NCT01898390
25. www.japanest-nippon.com/en/news/detail.php?id=88
27. National Institute on Deafness and Other Communication Disorders (NIDCD); www.nidcd.nih.gov/health/hearing/pages/older.aspx
28. National Institute on Deafness and Other Communication Disorders (NIDCD); www.nidcd.nih.gov/health/statistics/pages/quick.aspx
29. Devarajan K, Staecker H, Detamore MS. A review of gene delivery and stem cell based therapies for regenerating inner ear hair cells. J. Funct. Biomater. 2011; 2(3): 249–70.
30. Izumikawa M, Minoda R, Kawamoto K et al. Auditory hair cell replacement and hearing improvement by Atoh1 gene therapy in deaf mammals. Nat. Med. 2005; 11(3): 271–6.
31. www.clinicaltrials.gov/show/NCT02132130
32. Wong WL, Su X, Li X et al. Global prevalence of age-related macular degeneration and disease burden projection for 2020 and 2040: a systematic review and meta-analysis. Lancet Glob. Health 2014; 2(2): e106–116.
CrossRef
33. www.cdc.gov/visionhealth/basic_information/eye_disorders.htm
34. Friedman DS, O’Colmain BJ, Munoz B et al. Prevalence of age-related macular degeneration in the United States. Arch. Ophthalmol. 2014; 122(4): 564–72.
35. Schwartz SD, Regillo CD, Lam BL et al. Human embryonic stem cell-derived retinal pigment epithelium in patients with age-related macular degeneration and Stargardt’s macular dystrophy: follow-up of two open-label phase 1/2 studies. Lancet 2015; 385(9967): 509–16.
CrossRef
36. Song WK, Park KM, Kim HJ et al. Treatment of macular degeneration using embryonic stem cell-derived retinal pigment epithelium: preliminary results in Asian patients. Stem Cell Reports 2015; 4(5): 860–72.
CrossRef
37. www.clinicaltrials.gov/show/NCT01691261
38. www.ipscell.com/2015/07/firstipscstop/
39. www.ds-pharma.com/news/2013/20131202.html
40. Kagimoto HTS. The Path Forward. Cell & Gene Therapy Insights 2015; 1(1) dx.doi.org/10.18609.cgti.2015.004
41. www.clinicaltrials.gov/show/NCT01632527
42. Gould L, Abadir P, Brem H. Chronic wound repair and healing in older adults: current status and future research. Wound Repair Regen. 2015; 23(1): 1–13.
CrossRef
43. Centers for Disease Control and Prevention (CDC). www.cdc.gov/nchs/data/databriefs/db14.pdf
44. Margolis DJ, Bilker W, Santanna J et al. Venous leg ulcer: incidence and prevalence in the elderly. J. Am. Acad. Dermatol. 2002; 46(3): 381–86.
45. www.clinicaltrials.gov/show/NCT02375802
46. www.clinicaltrials.gov/show/NCT02322554
47. Offermans MP, Du Moulin MF, Hamers JP et al. Prevalence of urinary incontinence and associated risk factors in nursing home residents: a systematic review. Neurourol. Urodyn. 2009; 28(4): 288–294.
CrossRef
48. Xu D & Kane RL. Effect of urinary incontinence on older nursing home residents’ self-reported quality of life. J. Am. Geriatr. Soc. 2013; 61(9): 1473–81.
CrossRef
49. Dissaranan C, Cruz MA, Couri BM et al. Stem cell therapy for incontinence: where are we now? What is the realistic potential? Curr. Urol. Rep. 2011; 12(5): 336–44.
50. www.clinicaltrials.gov/show/NCT01893138
51. Hart ML, Izeta A, Herrera-Imbroda B et al. Cell Therapy for Stress Urinary Incontinence. Tissue Eng. Part B Rev. 2015; 21(4): 365–76.
CrossRef
52. Giberti C, Gallo F, Schenone M, Cortese P, Ninotta G. Stem Cell Therapy for Male Urinary Incontinence. Urol. Int. 2013; 90(3): 249–252.
CrossRef
53. Mitterberger M, Marksteiner R, Margreiter E et al. Myoblast and fibroblast therapy for post-prostatectomy urinary incontinence: 1-year followup of 63 patients. J. Urol. 2008; 179(1): 226–31.
54. Gotoh M, Yamamoto T, Kato M et al. Regenerative treatment of male stress urinary incontinence by periurethral injection of autologous adipose-derived regenerative cells: 1-year outcomes in 11 patients. Int. J. Urol. 2014; 21(3): 294–300.
CrossRef