Exploiting RNA activation for therapeutic applications
Cell Gene Therapy Insights 2015; 1(1), 14-18.
DOI: 10.18609/cgti.2015.003
Editorial
The next frontier in RNA biology is the advancement of RNA therapeutics and cellular reprogramming for unmet clinical needs. Since the discovery of post-transcriptional RNA interference (RNAi) by Fire and Mello, the RNA field has made tremendous progress, and small double-stranded RNAs (dsRNAs) play a pivotal role in our understanding of gene regulation. These small RNA molecules are known as non-coding RNAs (ncRNA) because they do not translate into biological proteins. The best classical examples and highly studied ncRNAs are microRNAs (miRNA) and small interfering RNAs (siRNA). They are essential in gene regulation through their ability to suppress target genes. Over the years, many other types of ncRNA have been identified such as long non-coding RNAs (lnc-RNA), Piwi-interacting RNA (piRNA), enhancer RNAs (eRNA), and extracellular RNAs (exRNA). This editorial will not cover these ncRNAs but only mention them in passing since they are already the subject of excellent published review articles. Rather, we will focus on another class of lesser known ncRNA called small/short activation RNAs (saRNA), or antigene RNAs (agRNA), due to their unique properties and controversial status. As the name suggests, these are short stretches of dsRNA molecules similar to siRNAs but with a unique ability to turn on genes rather than suppress them.
The term ‘RNA activation’ (RNAa) was coined by Li and colleagues to describe this kind of RNA activity, as well as to distinguish it from the RNAi pathway. SaRNAs are short synthetic 19-21-nucleotide dsRNAs, which target specific regulatory sequences, such as promoter and enhancer regions of genes, and function at the pre-transcriptional level. The mechanism is unknown but it has been speculated that RNA Pol II transcription machinery and the Argonaute proteins (Ago) may be involved. RNAa is evolutionally conserved in mammals and it may serve as a counter-balance to RNAi activity in cells.
Historically, RNAa was accidentally discovered by Long-Cheng Li and colleagues [1] when they were studying the E-cadherin promoter region using designed small dsRNAs by applying the siRNA rules to activate the RNAi pathway. To their surprise, they observed that the small dsRNA overexpressed E-cadherin instead of the expected down-regulation that is normally associated with siRNAs. They performed more studies to verify their findings and, as a result, they concluded that small dsRNAs could activate genes [2]. Since then other laboratories have also validated and reported RNAa using saRNAs and the list of target genes are growing (MAFA, CEBPA, HNF4-alpha, Oct4, Myc, KLF4, p21, E-cadherin, progesterone receptor, NKX3-1). The discovery of RNAa was exciting because it provided a more direct approach to upregulating genes (gain-of-function) without the time-consuming conventional method of engineering and designing expression vectors. It would also provide a powerful tool to manipulate gene expressions at the cellular level with minimal cost.
Unlike RNAi where the mechanism for the assembly of siRNA with the RNA-induced silencing complex (RISC) in the cytoplasm is well defined, the mechanism of saRNA assembly in the cell nucleus is unknown. However, there are reports to suggest that Argonaute proteins may be involved in the processing of saRNA assembly as demonstrated by knockdown experiments. A few studies have suggested that Ago–saRNA complex may function at the transcriptional level by forming a larger complex with RNA Pol II machinery in the nucleus [2,3]. This alliance may trigger epigenetic changes, including methylation and histone modifications, thereby allowing transcriptional activity to proceed. The shuttling of the Ago–saRNA complex from the cytoplasm to the nucleus is unknown and it remains to be determined which of the Argonaute proteins are essential in this process.
In addition to the subcellular localisation difference between RNAa and RNAi activity, there are other differences such as the time it takes to activate the pathway. For example, it takes RNAi only a few hours to demonstrate an effect, whereas RNAa activity can be seen within 24 to 48 hours. Another difference is the stability and duration of the dsRNA; for saRNAs this can range from 1 to 2 weeks compared to a few days for siRNAs and miRNAs. All ncRNAs including saRNAs suffer from a major problem – the off-target effect [2,4]
Unlike designing siRNAs for which software programs are readily available, saRNAs are designed manually and tested individually, which can be a huge challenge and drain on time. This is especially true for the testing of genes that have large promoters or if multiple genes are being investigated. In addition, there is the increased possibility of accidentally designing siRNAs and selecting poor saRNA candidates. Only recently, a couple of software programs have been developed for saRNAs to address these concerns and reduce the time to design saRNAs [5–8]. This has proven to be a much faster approach than manually selecting regions within the promotor regions. The software program is designed to examine the entire human genome using an automated genomic–bioinformatics approach [5-8]. Among many features, one unique parameter of the program is its ability to automatically exclude siRNA and miRNA sequences while generating the highest probability of identifying specific saRNA sequences targeting the promoter region for gene activation. This is particularly relevant because it will prevent overlapping with other ncRNA sequences, as well as providing a high through-put approach which takes advantage of the human genome database.
At present, no clinical trials have been reported using saRNAs. However, Dr Nagy Habib at Imperial College London and colleagues are developing a unique clinical grade saRNA molecule in collaboration with MiNA Therapeutics that could one day be delivered safely and efficiently for the treatment of liver disease. The group has shown that when saRNAs are injected into tumor-bearing animals the saRNA reduces the tumor burden significantly [7]. Increasingly, other investigators have also shown that saRNAs are effective and safe agents in various animal models [3,9].
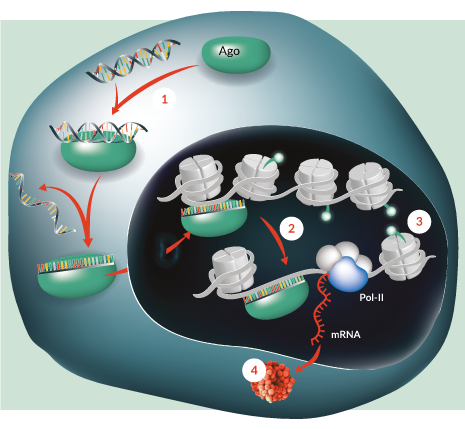
Figure 1. saRNA mechanism of action. 1. saRNA is loaded onto Argonaute proteins (Ago) in the cytosol and translocates into the nucleus. 2. saRNA–Ago complex promotes the recruitment of all endogenous transcription complexes, including RNA Polymerase II (Pol-II). 3. saRNA–Ago complex triggers promoter remodeling at the target promoter region 4. MiNA has demonstrated functional up-regulation of several proteins in a diverse range of cell types as well as in vivo. Image adapated from MiNA Therapeutics (http://minatx.com/science/)
In addition to using saRNAs as a potential therapeutic agent for the treatment of cancer, they are also being exploited for direct cellular re-programming for stem cell research and regenerative medicine. With the discovery of the induced pluripotent stem cells by Dr Shinya Yamanaka [10], the idea of reprogramming normal somatic cells into stem cells was revolutionary because it provided researchers with an alternative stem cell resource instead of relying on the controversial embryonic stem cells. However, it was later realized that the approach had technical issues regarding translatability and the high risk of tumor formation. The saRNA approach may provide an alternative path since reports have shown that the four Yamanaka genes in fact could be overexpressed using specific saRNAs. In addition, studies have demonstrated that saRNAs can be used to reprogramme somatic cells and their cell fate. For example, saRNA molecules against the pancreatic-specific gene promoter, MAFA, were used to reprogramme CD34+ cells into insulin-secreting cells [5]. Unlike RNAi, RNA activation is in its infancy and will require more investigation before it is fully appreciated. It does add another layer of complexity to the RNA world but an intriguing one that will someday provide a powerful direct approach to developing RNA therapeutics for the treatment of cancer, as well as increasing our understanding of the multi-faceted world of RNA.
This work is licensed under a Creative Commons Attribution- NonCommercial – NoDerivatives 4.0 International License.
Affiliations
Paul J Mintz,
Liverpool John Moores University, Faculty of Science, UK
Vikash Reebye
Imperial College London, Faculty of Medicine, Department of Surgery & Cancer, UK
Pål Sætrom,
Norwegian University of Science and Technology, NTN, Department of Computer and Information Science and Department of Cancer Research and Molecular Medicine Faculty of Medicine, Norway
John J Rossi
Beckman Research Institute of City of Hope, Molecular and Cellular Biology, USA
Nagy A Habib
Imperial College London, Faculty of Medicine, Department of Surgery & Cancer, UK
Financial & Competing Interests Disclosure
The authors have no relevant financial involvement with an organisation or entity with a financial interest in or financial conflict with the subject matter or materials discussed in the manuscript. This includes employment, consultancies, honoraria, stock options or ownership, expert testimony, grants or patents received or pending, or royalties.
No writing assistance was utilized in the production of this manuscript.
References
1. Li LC, Okino ST, Zhao H, Pookot D, Place RF, Urakami S, Enokida H, Dahiya R. Small dsRNAs induce transcriptional activation in human cells. Proc. Natl Acad Sci USA. 2006; 103(46): 17337-42.
CrossRef
2. Portnoy V, Huang V, Place RF, Li LC. Small RNA and transcriptional upregulation. Wiley Interdiscip. Rev. RNA. 2011; 2(5): 748-60.
Crossref
3. Janowski BA & Corey DR. Minireview: Switching on progesterone receptor expression with duplex RNA. Mol. Endocrinol. 2010; 24(12): 2243-52.
CrossRef
4. Fellmann C, Lowe SW. Stable RNA interference rules for silencing. Nat. Cell Biol. 2014; 16(1): 10-8.
CrossRef
5. Reebye V, Sætrom P, Mintz PJ et al. A Short-activating RNA Oligonucleotide Targeting the Islet β-cell Transcriptional Factor MafA in CD34 (+) Cells. Mol. Ther. Nucleic Acids 2013; 2: e97.
CrossRef
6. Voutila J, Sætrom P, Mintz P et al. Gene Expression Profile Changes After Short-activating RNA-mediated Induction of Endogenous Pluripotency Factors in Human Mesenchymal 7. Stem Cells. Mol. Ther. Nucleic Acids 2012; 1: e35.
CrossRef
8. Reebye V, Sætrom P, Mintz PJ et al. Novel RNA oligonucleotide improves liver function and inhibits liver carcinogenesis in vivo. Hepatology 2014; 59(1): 216-27.
CrossRef
9. Wang J, Place RF, Portnoy V et al. Inducing gene expression by targeting promoter sequences using small activating RNAs. J. Biol. Methods. 2015; 2(1), pii: e14.
CrossRef
10. Place RF, Wang J, Noonan EJ et al. Formulation of Small Activating RNA Into Lipidoid Nanoparticles Inhibits Xenograft Prostate Tumor Growth by Inducing p21 Expression. Mol. Ther. Nucleic Acids 2012; 1: e15.
CrossRef
11. Takahashi K & Yamanaka S. Induction of pluripotent stem cells from mouse embryonic and adult fibroblast cultures by defined factors Cell 2006; 126(4): 663–76.
CrossRef